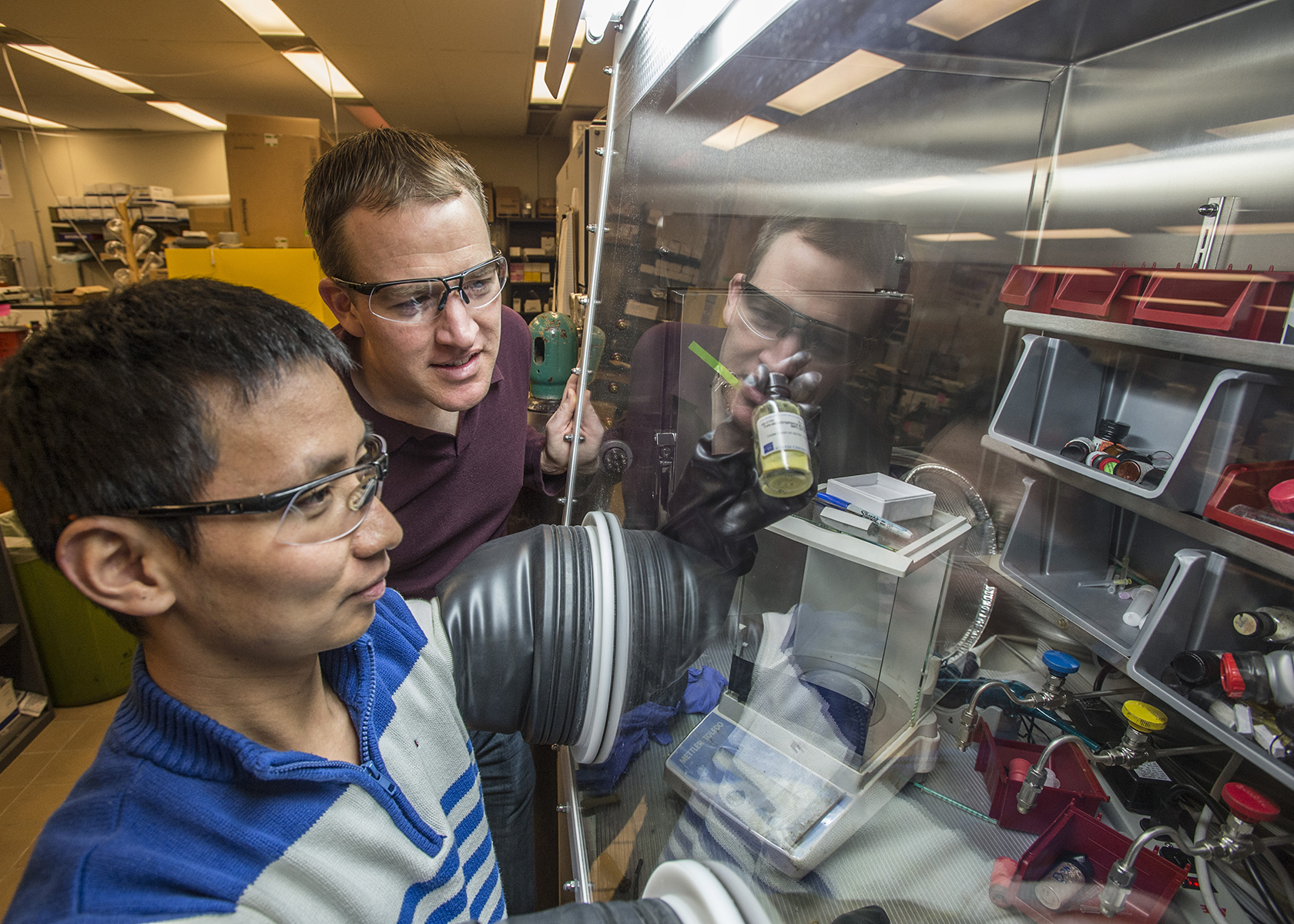
Find new ways to harness solar power -- Kyle Plunkett, center, assistant professor chemistry in the College of Science at Southern Illinois University Carbondale, along with Xinju Zhu, a doctoral student from is from Zhengzhou, China, use a glove box to work on materials in Plunkett’s chemistry laboratory. Plunkett and his team are finding ways to break down so-named “Buckminster Fullerenes,” soccer ball-shaped molecules known for the ability to accept free electrons under certain conditions in solar energy systems. Plunkett, who recently received a prestigious CAREER grant from the National Science Foundation, is doing fundamental research – making discoveries that formulate the basic building blocks of scientific knowledge. (Photo provided)
December 01, 2014
Solar energy research would make Fuller proud
CARBONDALE, Ill. -- A researcher at Southern Illinois University Carbondale is finding ways to tear down geodesic domes and use the parts in ways that have never been attempted.
But don’t worry, he’s working at the molecular level only. And R. Buckminster Fuller, the former SIU professor, design and geodesic dome guru and sustainability advocate, would likely very much approve of the effort, which has as its aim finding new ways to harness solar power.
Kyle Plunkett, an assistant professor of chemistry in the College of Science, along with his graduate researchers, are finding ways in the laboratory to break down so-named “Buckminster Fullerenes,” soccer ball-shaped molecules known for the ability to accept free electrons under certain conditions. The molecules, first created in a lab in 1985, are named after the world-famous Fuller, who taught at SIU from 1959 to 1970.
Plunkett, who recently received a prestigious CAREER grant from the National Science Foundation, is doing fundamental research – making discoveries that formulate the basic building blocks of scientific knowledge. But the ultimate goal is finding ways to improve solar energy conversion efficiencies in photovoltaic cells, otherwise known as solar cells. The basis of the research involves using organic-based materials, known as semiconductors. Semiconductors can transport electrons, though not as easily as copper or other metals.
The whole idea for such cells involves combining two different types of organic semi-conductors, one of which will give up electrons while the other tends to accept electrons. When the two materials are combined in a solar cell and then exposed to sunlight, electrons will flow from one region to the other, thus creating a current that can be stored as electricity in batteries as part of a solar power system.
“The compounds utilized as semiconductors tend to have large electron clouds,” Plunkett said. “The electrons located in these clouds can move within the molecular frameworks. They’re called delocalized electrons, in that instead of being isolated on a single atom, they can travel through a network of atoms.
“The larger that network is, sometimes you can start to get interesting properties such as more efficient charge transfer between molecules,” which can be beneficial for harnessing solar energy, he said.
Organic-based photovoltaic cells, however, are only about 10 percent efficient. Still, they maintain their potential as leading thrust in solar energy by virtue of their relative inexpensiveness and the ability to easily “print” them onto large surface areas quickly, much like a newspaper press prints on paper.
Increasing efficiency, therefore, is a priority. One way Plunkett’s lab is approaching that problem is by focusing on creating more efficient “acceptor” materials. That’s where the Buckminster Fullerene comes in.
Fullerenes are part of a family of compounds known as “cyclopenta-fused polycyclic aromatic hydrocarbon” – or a CP-PAH in chemistry parlance. Plunkett and his students use organic and synthetic chemistry to essentially take fullerenes apart and then use their pieces to make new and better materials that more readily promote electron flow in a semiconductor-based solar cells.
“Simplistically speaking, a lot of my lab’s work is about mixing starting materials in round-bottom flasks, letting it react overnight and then doing a lot of purification steps,” said Plunkett, whose NSF CAREER grant will provide $650,000 during its five-year life for his work in this area. “And we hopefully make these large conjugated systems.”
Buckminster Fullerenes, constructed of 60 carbon atoms, are the most widely used electron-accepting material in organic photovoltaic devices, Plunkett said. “So the very specific end of this is we are trying to replace the most-utilized electron acceptor out there,” he said. “There’s been a great deal of research into materials that give up electrons and not so much on materials that accept them.”
Once the fullerenes have been chemically disassembled, they can be reassembled in such a way that single and double bonds alternate in a network, aiding the transfer of electrons when sunlight shines on the cell and excites the electrons to a higher state of energy.
Plunkett’s team has been able to create several new versions or “targets” that show promise. All are similar in structure and they are making them in his lab. Several of them don’t project onto a fullerene and are completely different, though they still utilize a CP-PAH substructure.
“Right now we have a single framework we’re building on and we’re looking for larger and larger systems to enhance the electronic characteristics,” Plunkett said.
One reason the electrons want to flow is that fullerene is lower in energy than the donor material. Plunkett said that situation is necessary, but currently not optimal, resulting in lost efficiency. It’s a little like being on the top of a hill and trying to walk down and then up to the top of a neighboring hill, in that you want to find the path that will be easiest.
Using different chemical processes, his team is finding ways to “tune” the new accepting materials they are creating in an attempt to overcome this issue.
“The key is where there is an offset in energy between your donor and your acceptor. We want the acceptor to be lower in energy so the electron doesn’t mind hopping over there. But we don’t want it to be so much lower that we lose a lot of energy when we do this,” he said. “You want the acceptor to be high in energy, but lower than the donor material. So it’s all about tuning the materials. It’s the ability to tune that we want to achieve. We are going for the optimal offset in energy.”
Still, improving on the 10 percent efficiency that is the standard today perhaps remains a bit out of reach. But the fundamental research Plunkett and his team are doing in its pursuit could yield important information that someday unlocks solar power’s potential.
“The problem has been that efficiencies are not as good as you’d like,” he said. “The 10 percent is getting close. Some say if you could get to 12 or 15 percent, they would be commercially viable, at least in niche markets if not beyond. I don’t dream that we will ever get anywhere close to that, but our work is sort of a proof of principle that we can make other materials that can do this too.
“That’s why fundamental research is important. You might be able to find a very stable or useful molecule, or draw up some new theory that down the road makes the higher efficiency devices possible,” he said.